碳纤维复合材料胶粘剂设计和配置考虑事项
By www.carbonfiber.com.cn
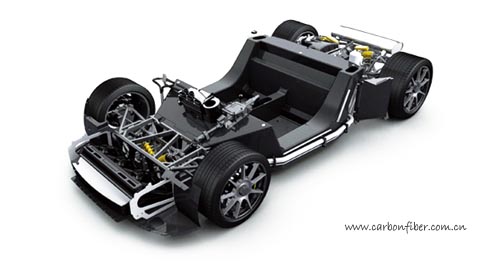
碳纤维复合材料车部件
如今,许多公司使用特定碳纤维复合材料来替代传统材料,包括使用结构型胶粘剂来连接不同材料。为了节省这种连接方式的成本,提高产品优异性能,设计师需考虑一系列新变量:接头设计/粘合剂装配, 要求装配时间, 表层准备/胶层厚度, 异种材料热膨胀, 成本效率,屈服应力和理想失效模式。
接头设计/胶粘剂装配
粘合接头设计时需要评估的第一个变量。粘合剂的有效性高度依赖于基片的表面,环境以及应用到接头的压力也会影响胶粘剂的有效性。
三种基本应力类型:张应力、剪切、剥离。
张应力
张应力仅应用于理想的装配应用领域。无任何剥离因素在内,压力或负荷延伸了统一方向的整个黏性面积。 这是很罕见的,因为胶接件容易弯曲,产生一些剥离压力。
剪切应力
另一个比较常见的应力是剪切应力。剪切应力测试将为设计者提供有用的信息。这种应力类型本身可直接导致粘合剂或基片(分层或破损)分离,穿过粘着力时,力量是向着相反的方向拉伸的。当连接两个扁平相似或不同基片时,可应用搭接处。
剥离应力
剥离应力是需要评估的最后一种类型应力。一般而言,测试时,使用刚性或柔性材料,可产生剥离应力。由于粘合物分离,传至粘合胶层,粘合接头一边的压力可出现故障。一般情况下,剥离强度比剪切强度要低,因为破损点局限于粘合剂的边缘,一旦出现故障,仅较少的压力就可导致故障逐渐延伸。因此,当胶接件对剪切应力起反应时,剥离应力消除后,产生了有效的粘合接头。如果无法避免剥离应力,可评估或选择更多具有持久性应用的剥离应力的柔性胶粘剂。
设计粘合接头时,需要考虑的主要设计准则包括:最大剪切/最小剥离和分裂;最大压缩/最小拉伸应力;接缝宽度比重叠长度重要得多。
装配时间
随着对大容量和高速产品需求不断增长,组件装配时间非常重要。需要回答的一个问题是,当使用胶粘剂时,将基片连接在一起,粘合接头固定后,多久可付诸使用?
为了达到这一目的,室温情况下,结构型胶粘剂工作实践也不同。为了确保胶粘剂有效性,需要考虑连接两个相似或不同材料的工作实践。这一段时间可能延长,也可能会缩短,主要依赖于所使用的粘合结构、周围温度和环境。一些两层结构型胶粘剂只有在室温情况下才能凝固,反应时间受温度的影响。一些快速凝固型粘合剂可将传统装配时间减少一半。
一旦确立了工作时间,设计师应知道或了解胶粘剂的发展强度。通过物理测试,可评定胶粘剂的强度。相对固定时间是,移动装配之前,夹紧或固定粘结接头时。在这段时间中,设计师应识别,使用胶粘剂,哪种当前装配程序可省略,装配需要多长时间,可节省多少时间。
表面处理/胶层厚度
与传统铆钉和紧固件相比,胶粘剂是一种比较有效的连接方法,因为物理负荷可分散到更宽阔的表面中去。设计的结构型胶粘剂具有优异的表面附着力,表面粘力非常高。然而,表面可能会变因为基础材料的化学性、模制或加工条件的变化,复合材料部分使用的脱模剂也会影响。
一些结构性胶粘剂规模较大,需要一定量的粘合材料才能起反应,适当凝固。所以,对设计师而言,标准的胶衣厚度非常重要。通过几种方法可获得适宜的厚度。
对于浇铸型复合材料部件而言,粘结之前,可设计胶衣厚度。如果模子内无法计算出胶粘剂胶衣的公差,简单测量一下即可。可使用一些较小的塑胶垫片或薄型塑料垫片来确定厚度。
当相似或不同材质中增加压力时,压力应足够明显,可清晰看到粘合面积两端中粘合剂的流动。如果粘合接头处使用了过度的压力,制造商就要承担“缺少”胶衣的风险。当大部分胶粘剂从粘合面积中被挤出来时,缺少胶衣的情况就会发生。缺少胶衣的结果一般是粘结接头效果较弱,缺乏全部黏性物理属性。
热膨胀
另一个需要考虑的设计变量是热膨胀系数的不同,尤其是连接两个不同材料时。由于其不同密度,两种物质接受和传输的热量不同。粘合温度和环境条件也会有区别。因此,热膨胀系数是常数项变量,
不应忽略这一因素。然而,当使用具有较大灵活性和延伸率的结构型胶粘剂时,这一常数项变量将不再是一个问题。选择了合适胶粘剂,不同材料暴露于不同温度条件下时,凝固了的结构型胶粘剂可抵抗和抵消产生的压力。
成本效率
最终装配的强度依赖于接头设计、胶粘剂系统的有效性,物理负荷是如何分散至装配线的。如需回答物理负荷是如何分散的,设计师应将胶粘剂覆盖范围最大化。此外,胶粘剂接头应具有最大的表面面积。
结合在一起时,胶粘剂覆盖面或表面尺寸的数量可影响装配的强度以及承受较大负荷的能力。表面面积越大,胶粘剂强度也就越大。表面面积取决于胶粘剂覆盖面的宽度和时间长短。厚度或高度取决于胶衣厚度,结构型胶粘剂应先确定胶衣厚度。统一的胶衣厚度非常重要,有助于测量胶粘剂的性能。
当使用结构型胶粘剂替代传统胶粘技术时,必须进行以下计算,以确保设计的精确性。
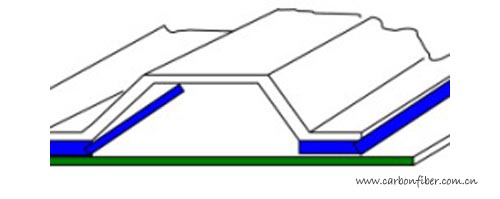
复合材料装配
图1为复合材料装配的法兰。在这一案例中,考虑一下25毫米宽的法兰,具有恒定的胶衣厚度。至于这方面的研究,我们将考虑法兰宽度的变化。这些计算是建立在法兰宽度变化的基础之上的,因为对复合材料制造者而言,改变模具法兰尺寸比改变控制胶衣厚度的模具公差要容易地多。在承载重量保持不变的情况下,结构型胶粘剂可节省成本。
屈服应力和理想失效模式
最后一项设计变量是验证胶接件的屈服应力和实效模式。胶粘剂合格和在目前应用领域应用的要求是什么呢?胶接处的物理测试要求分析和确认实效模式。对设计师或生产商而言,实效模式结构和压力分析所得结果价值非常大。这一信息可作为最终装载负荷要求的基准。
在某种意义上,测试胶接处的关键是寻找类似于实际胶接件的产品。测试一旦完成,可评估失效模式。一般情况下,黏性测试失效由胶粘剂、粘聚性以及基片实效模式组成。其它失效模式确实存在,但都和表面有关系。在此程序中以及早期阶段,经常使用工程软件程序,以帮助识别高应力区。
原文来源:塑料树脂
Today, many companies are replacing traditional methods of fixing carbon fiber composite materials – including the joining of dissimilar materials – with processes involving the use of structural adhesives. In order to enjoy the cost and production benefits of this bonding method, designers need to take into account a new set of variables:
Joint design/bond assembly,required assembly time, surface preparation/bondline thickness, thermal expansion of dissimilar materials, cost effectiveness; and yield stress and desirable failure mode.
Joint design/bond assembly
Adhesive joint design is the first variable to evaluate. Effective bonding is highly dependent on the surface of the substrate, the environment, and understanding the stresses applied to the joint.
The three basic types or stresses are: tensile, shear and peel.
Tensile stress
Tensile stress is applied only in an ideal assembly application. Forces or loads are elongating the entire adhesive area in a uniform direction without any element of peel. This is rare since the nature of the bonded assembly will tend to bend and flex, creating some peel stresses.
Shear stress
Another common stress is that due to shear. Shear stress testing will provide useful information for the designer. This stress type will inherently cause separation in either the adhesive or the substrate (delamination or breakage) because forces are pulling in opposing directions across the adhesive bond. Lap joints are applicable when bonding two flat similar or dissimilar substrates.
Peel stress
Peel stress is the last stress type to evaluate. Typically, peel stresses can generate within rigid or flexible substrate materials when tested. Forces at one edge of the bonded joint can cause failures due to the adhesive separating and propagating across the adhesive bondline. Peel strengths are typically lower than shear strengths because the point of failure is limited to the edge of the bond and less force is required to propagate a failure once initiated. Hence, an effective adhesive joint is created when the bonded assembly is reacting to shear stresses while peel stresses are eliminated. If peel stresses cannot be avoided, evaluate or choose a more flexible adhesive with the capability of enduring applied peel stresses.
The main design guidelines to be considered when designing an adhesive joint include:
Maximise shear/minimise peel and cleavage; maximise compression/minimise tensile; and joint width is more important than overlap length.
Assembly time
With increasing demand for high volume and high speed production, the component assembly time is critical. One question that has to be answered when using an adhesive is how much time is required for applying the adhesive, joining the substrates, and clamping the bonded joint?
Structural adhesives will offer various working times at room temperature to achieve this goal. Working time is considered the period of time one has to join two similar or dissimilar materials to ensure an effective bond.
This period of time may increase or decrease depending on the adhesive system used, ambient temperature and the environment. Some two-part structural adhesives are room-temperature cured products and the reaction time is affected by temperature. Faster fixture time adhesives can cut some conventional assembly times in half.
Once the working time is established, the designer should know or understand the developmental strength of the adhesive. This can be assessed through physical testing. The relative fixture time is the period of time for which the bonded joint needs to be clamped or fixed prior to moving the assembly. By this stage, the designer should recognise which current assembly procedures can be eliminated by the use of an adhesive, and how much time is saved or gained in assembly.
Surface preparation/bondline thickness
Adhesives are an effective joining method since more physical loading can be distributed across a broader surface area in comparison to conventional rivets and fasteners.
Structural adhesives are designed for excellent surface adhesion and bonding is highly surface dependent. However, surfaces may change due to the chemistry of the base material, the surface contaminants, the moulding or processing conditions, and the release agents used in the moulding of composite parts.
Some structural adhesives are mass dependent and require enough adhesive material to react and cure properly. Consequently a nominal bondline thickness is advantageous to a designer. There are a few ways to create a bondline thickness.
For moulded composite parts, bondline thickness can be created prior to bonding through designed presets in the mould with stand-offs or ’dimples.’If there is no feasibility to create a tolerance for an adhesive bondline within the mould, then simple measures can be just as efficient. Small plastic washers or thin plastic spacers can be used to establish some thickness.’
When applying pressure to join similar or dissimilar materials, pressure should be significant enough to visibly see adhesive flowing from the sides of the bond area. If excessive pressure is applied to the bonded joint without any bondline thickness controls, the manufacturer can run the risk of ’starving’ the bondline. This occurs when the majority of the adhesive is squeezed out of the bond area without a significant amount of mass to cure properly. The result of starving a bondline is usually a weak bonded joint that lacks the full adhesive physical properties.
Thermal expansion
Another design variable to consider, especially when bonding two dissimilar materials, is the difference in thermal expansion. How the two materials accept and transfer heat will be different, due to their different densities. Bonding temperatures and environmental conditions may always vary. So, the coefficient of thermal expansion is a constant variable that should not be ignored. However, this constant usually is less of an issue when using structural adhesives with high flexibility and elongation. When the proper adhesive is selected, the cured structural adhesive will be able to resist and compensate for the stresses created when dissimilar materials are exposed to various temperature conditions.
Cost effectiveness
When bonding, the amount of adhesive coverage or size of surface area will affect the strength of the assembly and its ability to accept higher physical loads. The greater the surface area, the greater the bond strength. The surface area will be based on the length times the width of the adhesive coverage. A depth or height based on bondline thickness should be established for a structural adhesive. A uniform bondline thickness can be critical and will assist in gauging adhesive performance.
The strength of the final assembly will depend on an effective joint design, adhesive system, and how well physical loads are distributed across the assembly. To answer how well physical loads are distributed, the designer should maximise adhesive coverage. Also, the adhesive joint design should allow for maximum surface area.
As an example, consider a flange on a composite assembly shown in Figure 1. For this case, consider a 25 mm wide flange with constant bondline thickness. As far as this study is concerned, we will consider changes in the flange width. These computations are based on width changes (flange width), since it is easier for a composite fabricator to change the mould flange dimensions than it is to change mould tolerances to control bondline thickness. Structural adhesives can reduce costs by allowing bond areas to be reduced whilst maintaining the same load bearing capabilities.
When replacing conventional joining techniques with structural adhesives, the following calculations must be performed to ensure the design would be adequate.
Yield stress and desirable failure mode
One of the last joint design variables to verify is yield stresses of the bonded assembly and failure modes. What is required to qualify this adhesive and implement it to the current application? Physical testing on the bonded joint is required to analyse and confirm the mode of failure. Resulting failure modes and stress values achieved are valuable tools for the designer or manufacturer. This information will serve as a benchmark for final assembly load requirements.
The key is to test the bonded joint in a manner that resembles the actual bonded assembly. Once tested, failure modes can be evaluated. Generally, adhesive test failures will consist of adhesive, cohesive, and substrate failure modes. Other failure modes do exist, but are surface related. Engineering software programs are often used in this process as well as earlier stages to assist in identifying high stressed areas. They can also design in appropriate variables such as substrate thickness.
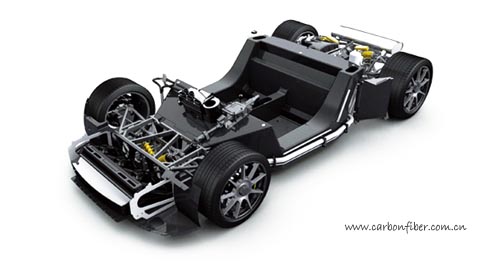
碳纤维复合材料车部件
如今,许多公司使用特定碳纤维复合材料来替代传统材料,包括使用结构型胶粘剂来连接不同材料。为了节省这种连接方式的成本,提高产品优异性能,设计师需考虑一系列新变量:接头设计/粘合剂装配, 要求装配时间, 表层准备/胶层厚度, 异种材料热膨胀, 成本效率,屈服应力和理想失效模式。
接头设计/胶粘剂装配
粘合接头设计时需要评估的第一个变量。粘合剂的有效性高度依赖于基片的表面,环境以及应用到接头的压力也会影响胶粘剂的有效性。
三种基本应力类型:张应力、剪切、剥离。
张应力
张应力仅应用于理想的装配应用领域。无任何剥离因素在内,压力或负荷延伸了统一方向的整个黏性面积。 这是很罕见的,因为胶接件容易弯曲,产生一些剥离压力。
剪切应力
另一个比较常见的应力是剪切应力。剪切应力测试将为设计者提供有用的信息。这种应力类型本身可直接导致粘合剂或基片(分层或破损)分离,穿过粘着力时,力量是向着相反的方向拉伸的。当连接两个扁平相似或不同基片时,可应用搭接处。
剥离应力
剥离应力是需要评估的最后一种类型应力。一般而言,测试时,使用刚性或柔性材料,可产生剥离应力。由于粘合物分离,传至粘合胶层,粘合接头一边的压力可出现故障。一般情况下,剥离强度比剪切强度要低,因为破损点局限于粘合剂的边缘,一旦出现故障,仅较少的压力就可导致故障逐渐延伸。因此,当胶接件对剪切应力起反应时,剥离应力消除后,产生了有效的粘合接头。如果无法避免剥离应力,可评估或选择更多具有持久性应用的剥离应力的柔性胶粘剂。
设计粘合接头时,需要考虑的主要设计准则包括:最大剪切/最小剥离和分裂;最大压缩/最小拉伸应力;接缝宽度比重叠长度重要得多。
装配时间
随着对大容量和高速产品需求不断增长,组件装配时间非常重要。需要回答的一个问题是,当使用胶粘剂时,将基片连接在一起,粘合接头固定后,多久可付诸使用?
为了达到这一目的,室温情况下,结构型胶粘剂工作实践也不同。为了确保胶粘剂有效性,需要考虑连接两个相似或不同材料的工作实践。这一段时间可能延长,也可能会缩短,主要依赖于所使用的粘合结构、周围温度和环境。一些两层结构型胶粘剂只有在室温情况下才能凝固,反应时间受温度的影响。一些快速凝固型粘合剂可将传统装配时间减少一半。
一旦确立了工作时间,设计师应知道或了解胶粘剂的发展强度。通过物理测试,可评定胶粘剂的强度。相对固定时间是,移动装配之前,夹紧或固定粘结接头时。在这段时间中,设计师应识别,使用胶粘剂,哪种当前装配程序可省略,装配需要多长时间,可节省多少时间。
表面处理/胶层厚度
与传统铆钉和紧固件相比,胶粘剂是一种比较有效的连接方法,因为物理负荷可分散到更宽阔的表面中去。设计的结构型胶粘剂具有优异的表面附着力,表面粘力非常高。然而,表面可能会变因为基础材料的化学性、模制或加工条件的变化,复合材料部分使用的脱模剂也会影响。
一些结构性胶粘剂规模较大,需要一定量的粘合材料才能起反应,适当凝固。所以,对设计师而言,标准的胶衣厚度非常重要。通过几种方法可获得适宜的厚度。
对于浇铸型复合材料部件而言,粘结之前,可设计胶衣厚度。如果模子内无法计算出胶粘剂胶衣的公差,简单测量一下即可。可使用一些较小的塑胶垫片或薄型塑料垫片来确定厚度。
当相似或不同材质中增加压力时,压力应足够明显,可清晰看到粘合面积两端中粘合剂的流动。如果粘合接头处使用了过度的压力,制造商就要承担“缺少”胶衣的风险。当大部分胶粘剂从粘合面积中被挤出来时,缺少胶衣的情况就会发生。缺少胶衣的结果一般是粘结接头效果较弱,缺乏全部黏性物理属性。
热膨胀
另一个需要考虑的设计变量是热膨胀系数的不同,尤其是连接两个不同材料时。由于其不同密度,两种物质接受和传输的热量不同。粘合温度和环境条件也会有区别。因此,热膨胀系数是常数项变量,
不应忽略这一因素。然而,当使用具有较大灵活性和延伸率的结构型胶粘剂时,这一常数项变量将不再是一个问题。选择了合适胶粘剂,不同材料暴露于不同温度条件下时,凝固了的结构型胶粘剂可抵抗和抵消产生的压力。
成本效率
最终装配的强度依赖于接头设计、胶粘剂系统的有效性,物理负荷是如何分散至装配线的。如需回答物理负荷是如何分散的,设计师应将胶粘剂覆盖范围最大化。此外,胶粘剂接头应具有最大的表面面积。
结合在一起时,胶粘剂覆盖面或表面尺寸的数量可影响装配的强度以及承受较大负荷的能力。表面面积越大,胶粘剂强度也就越大。表面面积取决于胶粘剂覆盖面的宽度和时间长短。厚度或高度取决于胶衣厚度,结构型胶粘剂应先确定胶衣厚度。统一的胶衣厚度非常重要,有助于测量胶粘剂的性能。
当使用结构型胶粘剂替代传统胶粘技术时,必须进行以下计算,以确保设计的精确性。
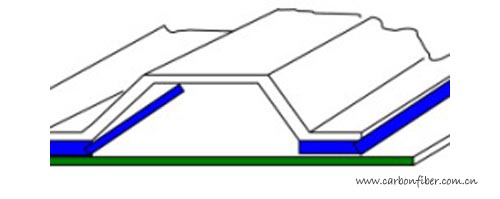
复合材料装配
图1为复合材料装配的法兰。在这一案例中,考虑一下25毫米宽的法兰,具有恒定的胶衣厚度。至于这方面的研究,我们将考虑法兰宽度的变化。这些计算是建立在法兰宽度变化的基础之上的,因为对复合材料制造者而言,改变模具法兰尺寸比改变控制胶衣厚度的模具公差要容易地多。在承载重量保持不变的情况下,结构型胶粘剂可节省成本。
屈服应力和理想失效模式
最后一项设计变量是验证胶接件的屈服应力和实效模式。胶粘剂合格和在目前应用领域应用的要求是什么呢?胶接处的物理测试要求分析和确认实效模式。对设计师或生产商而言,实效模式结构和压力分析所得结果价值非常大。这一信息可作为最终装载负荷要求的基准。
在某种意义上,测试胶接处的关键是寻找类似于实际胶接件的产品。测试一旦完成,可评估失效模式。一般情况下,黏性测试失效由胶粘剂、粘聚性以及基片实效模式组成。其它失效模式确实存在,但都和表面有关系。在此程序中以及早期阶段,经常使用工程软件程序,以帮助识别高应力区。
原文来源:塑料树脂
Today, many companies are replacing traditional methods of fixing carbon fiber composite materials – including the joining of dissimilar materials – with processes involving the use of structural adhesives. In order to enjoy the cost and production benefits of this bonding method, designers need to take into account a new set of variables:
Joint design/bond assembly,required assembly time, surface preparation/bondline thickness, thermal expansion of dissimilar materials, cost effectiveness; and yield stress and desirable failure mode.
Joint design/bond assembly
Adhesive joint design is the first variable to evaluate. Effective bonding is highly dependent on the surface of the substrate, the environment, and understanding the stresses applied to the joint.
The three basic types or stresses are: tensile, shear and peel.
Tensile stress
Tensile stress is applied only in an ideal assembly application. Forces or loads are elongating the entire adhesive area in a uniform direction without any element of peel. This is rare since the nature of the bonded assembly will tend to bend and flex, creating some peel stresses.
Shear stress
Another common stress is that due to shear. Shear stress testing will provide useful information for the designer. This stress type will inherently cause separation in either the adhesive or the substrate (delamination or breakage) because forces are pulling in opposing directions across the adhesive bond. Lap joints are applicable when bonding two flat similar or dissimilar substrates.
Peel stress
Peel stress is the last stress type to evaluate. Typically, peel stresses can generate within rigid or flexible substrate materials when tested. Forces at one edge of the bonded joint can cause failures due to the adhesive separating and propagating across the adhesive bondline. Peel strengths are typically lower than shear strengths because the point of failure is limited to the edge of the bond and less force is required to propagate a failure once initiated. Hence, an effective adhesive joint is created when the bonded assembly is reacting to shear stresses while peel stresses are eliminated. If peel stresses cannot be avoided, evaluate or choose a more flexible adhesive with the capability of enduring applied peel stresses.
The main design guidelines to be considered when designing an adhesive joint include:
Maximise shear/minimise peel and cleavage; maximise compression/minimise tensile; and joint width is more important than overlap length.
Assembly time
With increasing demand for high volume and high speed production, the component assembly time is critical. One question that has to be answered when using an adhesive is how much time is required for applying the adhesive, joining the substrates, and clamping the bonded joint?
Structural adhesives will offer various working times at room temperature to achieve this goal. Working time is considered the period of time one has to join two similar or dissimilar materials to ensure an effective bond.
This period of time may increase or decrease depending on the adhesive system used, ambient temperature and the environment. Some two-part structural adhesives are room-temperature cured products and the reaction time is affected by temperature. Faster fixture time adhesives can cut some conventional assembly times in half.
Once the working time is established, the designer should know or understand the developmental strength of the adhesive. This can be assessed through physical testing. The relative fixture time is the period of time for which the bonded joint needs to be clamped or fixed prior to moving the assembly. By this stage, the designer should recognise which current assembly procedures can be eliminated by the use of an adhesive, and how much time is saved or gained in assembly.
Surface preparation/bondline thickness
Adhesives are an effective joining method since more physical loading can be distributed across a broader surface area in comparison to conventional rivets and fasteners.
Structural adhesives are designed for excellent surface adhesion and bonding is highly surface dependent. However, surfaces may change due to the chemistry of the base material, the surface contaminants, the moulding or processing conditions, and the release agents used in the moulding of composite parts.
Some structural adhesives are mass dependent and require enough adhesive material to react and cure properly. Consequently a nominal bondline thickness is advantageous to a designer. There are a few ways to create a bondline thickness.
For moulded composite parts, bondline thickness can be created prior to bonding through designed presets in the mould with stand-offs or ’dimples.’If there is no feasibility to create a tolerance for an adhesive bondline within the mould, then simple measures can be just as efficient. Small plastic washers or thin plastic spacers can be used to establish some thickness.’
When applying pressure to join similar or dissimilar materials, pressure should be significant enough to visibly see adhesive flowing from the sides of the bond area. If excessive pressure is applied to the bonded joint without any bondline thickness controls, the manufacturer can run the risk of ’starving’ the bondline. This occurs when the majority of the adhesive is squeezed out of the bond area without a significant amount of mass to cure properly. The result of starving a bondline is usually a weak bonded joint that lacks the full adhesive physical properties.
Thermal expansion
Another design variable to consider, especially when bonding two dissimilar materials, is the difference in thermal expansion. How the two materials accept and transfer heat will be different, due to their different densities. Bonding temperatures and environmental conditions may always vary. So, the coefficient of thermal expansion is a constant variable that should not be ignored. However, this constant usually is less of an issue when using structural adhesives with high flexibility and elongation. When the proper adhesive is selected, the cured structural adhesive will be able to resist and compensate for the stresses created when dissimilar materials are exposed to various temperature conditions.
Cost effectiveness
When bonding, the amount of adhesive coverage or size of surface area will affect the strength of the assembly and its ability to accept higher physical loads. The greater the surface area, the greater the bond strength. The surface area will be based on the length times the width of the adhesive coverage. A depth or height based on bondline thickness should be established for a structural adhesive. A uniform bondline thickness can be critical and will assist in gauging adhesive performance.
The strength of the final assembly will depend on an effective joint design, adhesive system, and how well physical loads are distributed across the assembly. To answer how well physical loads are distributed, the designer should maximise adhesive coverage. Also, the adhesive joint design should allow for maximum surface area.
As an example, consider a flange on a composite assembly shown in Figure 1. For this case, consider a 25 mm wide flange with constant bondline thickness. As far as this study is concerned, we will consider changes in the flange width. These computations are based on width changes (flange width), since it is easier for a composite fabricator to change the mould flange dimensions than it is to change mould tolerances to control bondline thickness. Structural adhesives can reduce costs by allowing bond areas to be reduced whilst maintaining the same load bearing capabilities.
When replacing conventional joining techniques with structural adhesives, the following calculations must be performed to ensure the design would be adequate.
Yield stress and desirable failure mode
One of the last joint design variables to verify is yield stresses of the bonded assembly and failure modes. What is required to qualify this adhesive and implement it to the current application? Physical testing on the bonded joint is required to analyse and confirm the mode of failure. Resulting failure modes and stress values achieved are valuable tools for the designer or manufacturer. This information will serve as a benchmark for final assembly load requirements.
The key is to test the bonded joint in a manner that resembles the actual bonded assembly. Once tested, failure modes can be evaluated. Generally, adhesive test failures will consist of adhesive, cohesive, and substrate failure modes. Other failure modes do exist, but are surface related. Engineering software programs are often used in this process as well as earlier stages to assist in identifying high stressed areas. They can also design in appropriate variables such as substrate thickness.