汽车轻量化:可再生的低成本碳纤维制造底特律研讨会总结报告
By www.carbonfiber.com.cn
美国能源部:可再生低成本碳纤维制造研讨会总结报告

导语
2013年6月4-5日,美国能源部(DEO)下属的再生能源与能效办公室(EERE)在汽车城底特律举办了一场国际研讨会。会议以服务汽车轻量化为出发点,探讨了可再生、低成本碳纤维的研发和制造。参加会议的既有产业界的精英,也有学术界的权威专家。会议的主要议题包括如何寻找合适的可再生前驱体、如何将其应用于碳纤维生产并最终服务于汽车轻量化。
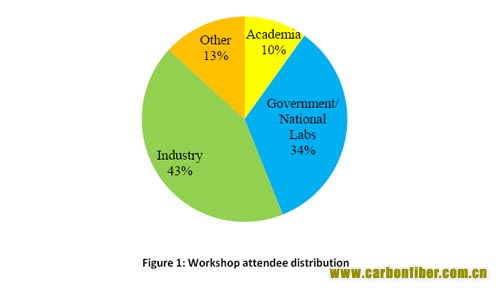
参会人员分类:产业界:43%;政府/国家实验室:34%;学校/研究院:10%;其他:13%
目的和议程
清洁能源生产动议书
美国能源部2013年7月间发布清洁能源生产动议书(Clean Energy Manufacturing Initiative),旨在提升美国在清洁能源生产领域的国际竞争力,进而借此提升美国制造产业的国际竞争力。该动议书由EERE下属的清洁能源技术办公室(Clean Energy Technology Office)和先进制造技术办公室(Advanced Manufacturing Office)负责具体操作,汇集了大批的产业界、学术界和政界的人员并机构,为美国制造业赢得未来市场的主动权谋划蓝图、制定战略。
为提升该动议书的影响力,美国能源部举办了一系列的座谈会、研讨会、咨询会,投资说明会等活动。作为系列会议之一,由能源部下属生物能源技术办公室(Bioenergy Technologies Office)与先进制造技术办公室(Advanced Manufacturing Office)、汽车技术办公室(Vehicle Technologies Office)合作举办了“汽车轻量化——可再生的低成本碳纤维制造”研讨会。研讨会的目的在于利用EERE及其下属各办公室的资源和专业优势,推动美国国内低成本碳纤维整个供应链条的技术进步,进而提升能源利用效率,提振美国制造业的国际竞争力。
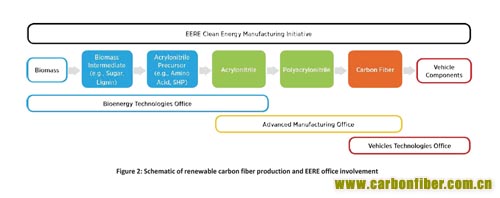
生物质资源——生物质中间体(糖类、木质素等)——丙烯腈前驱体(氨基酸、SHP等)——丙烯腈——聚丙烯腈——碳纤维——碳纤维汽车零部件
生物能源技术办公室(Bioenergy Technologies Office)
找到成本适宜、加工技术成熟的原材料(feedstock),并建立灵活稳定的供应链条是制造业成功的关键。对于碳纤维制造业来说,生物质资源(Biomass)充分满足上述条件,是一种可用以商业化运作的低成本原材料。在美国使用的所有可再生能源中,生物质能源毫无疑问是产量最大的一个。美国拥有世界上最大的耕地面积(约170万平方公里),其大豆、玉米灯农作物产量高居世界首位。美国同时是世界上最大的软木和硬木生产商。美国能源部最新发布的报告显示,全美农、林业生产剩余物(residue)等可再生生物资源多达10亿吨(干重),其中仅农作物生产剩余物一项(超过1.3亿吨),即便资源得不到充分转化,也远远超过碳纤维生产当今以及短期未来的需要。由于未经加工的生物质资源能量密度(Energy Density)较低,比较经济的方法是就近利用,实现碳纤维或其原丝的本地化生产。生物能源技术办公室的职责就在于想办法将国内可利用的生物质资源有效地转化成燃料、化学产品和电力能源。
先进制造技术办公室(Advanced Manufacturing Office)
该办公室的职能在于为橡树岭国家实验室(Oak Ridge National Laboratory, ORNL)的碳纤维技术试验生产线(Carbon Fiber Technology Facility, CFTF)提供研发支持。在CFTF中,研究人员主要从事包括木质素在内的多途径碳纤维前驱体制造技术的开发研究。单就木质素这一途径而言,ORNL已经取得了不小的进展。然而,在诸如汽车轻量化等具体应用中,使用木质素基碳纤维还存在一定的生产障碍。现如今想要实现木质素基碳纤维的商业化生产之前必须先解决以下几点困难:1. 熔融加工性能(Melt-Processability,尤其是软木木质素);2. 过长的热处理时间(尤其是硬木木质素);3. 原料(feedstock)庞杂,需要提纯(Purification)和分馏(Fractionation);4. 成丝后强度和硬度达不到预期指标;5. 规模量产可到什么程度未经验证。而其他前驱体的制备也存在类似的问题,比如说聚烯烃(Polyofelins)。以生物质资源为途径生产当今商业使用的PAN原丝的同等品,能使得上述障碍中的部分最小化,但是又会带了新的问题,好比说如何保证丙烯腈(Acrylonitrile)的纯度和质量。先进制造技术办公室的职能就是推动研发不同种前驱体材料的制备技术、降低产品生命周期内的能源消耗。该办公室同样推动研发能源转化技术和碳纤维制品的制造技术。
汽车技术办公室(Vehicle Technologies Office)
汽车轻量化是EERE的重要战略目标。汽车技术办公室的职能在于研究分析材料性能和制备工艺、推动研发新一代轻质材料。将碳纤维推广到量产车生产是一个耗资巨大的工程。据推算,只有当其使用成本降低到5美元/磅或以下的时候才可能实现。有鉴于此,研发出低成本的碳纤维制备方法将大大加快这一进程。轻质材料将使车重减轻50%,能耗降低35%左右,而其包括安全在内的各项性能并不受重量减轻的影响。美国能源部预测,使用碳纤维最多可减轻车重70%。即便车重仅仅降低10%,都会带来能耗4%-5%降低的红利。如今,小汽车每天要消耗880万桶石油。所有车重减轻10%,仅在美国每天就可以节约超过50亿加仑的汽油。
当下的技术状况
当下的碳纤维制备主要依赖PAN原丝,而PAN原丝炼自石油。PAN原丝的制备成本占到碳纤维整个制备成本的50%。石油精炼后的副产品丙烯(Propylene)经过催化氨氧化反应(Catalytic Ammoxidation)生成丙烯腈,再经过自由基聚合(Free Radical Polymerization)之后生成PAN原丝。因此,PAN原丝的价格受制于原油价格的波动,彼涨此涨。如若能找到PAN原丝的替代产品,碳纤维的使用成本必回大大下降。这种替代产品就是生物质资源。而美国能源部正在极力促成下属各办公室之间的合作来寻找这种合适的PAN原丝替代品。
研讨会的进程
为了让与会者有尽可能大的收获,美国能源部邀请了在碳纤维制造、生物质资源处理、中间体制备以及工业化学品生产领域经验丰富的专家学者发表演讲。这些专家学者将从各自专业领域的角度分析使用可再生碳纤维所需的满足的条件以及可能带来的好处。讲演的主题涵盖了碳纤维制备的技术挑战、应用于汽车零部件生产需要满足的条件、将生物质资源转化成碳纤维制备中间体材料的技术挑战以及非PAN基碳纤维带来的种种机遇等等。
在全体人员会议上,美国能源部将着重强调其对清洁能源制造技术和其他新技术的兴趣,介绍人包括:
·战略规划项目组 Elizabeth(Libby)Wayman
·生物能源技术办公室 Neil Rossmeissl
·先进制造技术办公室 Bhima Sastri
发表主题演讲的各领域专家学者包括:
·Plasan Carbon Composites公司 Gary Lownsdale
·橡树岭国家实验室 Cliff Eberle 和 Mark Downing
·Old Town Fuel & Fiber公司 Darrell Waite
·ADM公司 Will Schroeder
此外,研讨会还就三个议题分别设立了子会议。这是议题分别是:1. 碳纤维制备的技术挑战以及生物源材料制备汽车零部件需要满足的条件;2. 利用生物质资源生产低成本碳纤维中间体的技术挑战;3. 突破传统的碳纤维:资源、挑战和机遇。整个研讨会的时程安排详见原文。在子会议中,与会者将被分为三个小组,就一系列问题展开讨论(问题列表详见原文)。讨论内容将被记录、汇总并形成书面报告。
与会名单

注释
Plasan Carbon Composites公司:美国碳纤维汽车零部件生产企业
Old Town Fuel & Fiber公司:美国纸浆生产企业
ADM公司:世界最大的小麦、玉米及油籽加工企业之一
赞助单位:美国能源部 再生能源与能效办公室
生物能源技术办公室
报告整理:Joyce Yang Katy Christiansen Sarah Luchner
编译:中国复材展组委会
Renewable, Low‐Cost Carbon Fiber for Lightweight Vehicles
Summary Report from the June 4–5, 2013, Workshop
Detroit, MI
Workshop and Summary Report sponsored by the
U.S. Department of Energy
Office of Energy Efficiency and Renewable Energy
Bioenergy Technologies Office
Summary report edited by Joyce Yang, Katy Christiansen, and Sarah Luchner
Preface
The U.S. Department of Energy’s (DOE’s) Office of Energy Efficiency and Renewable Energy (EERE) invests in a diverse portfolio of energy technologies to achieve a stronger economy, a cleaner environment, and a more secure energy future for America.
This report summarizes the results of a public workshop sponsored by DOE/EERE in Detroit, Michigan, on June 4–5, 2013. The views and opinions of the workshop attendees, as summarized in this document, do not necessarily reflect those of the United States government or any agency thereof, or do their employees make any warranty, expressed or implied, or assume any liability or responsibility for the accuracy, completeness, or usefulness of any information, apparatus, product, or process disclosed, or represent that its use would not infringe privately owned rights.
Table of Contents
Introduction ...................................................................................................
Purpose, Need, and Process of the Workshop ...................................................................................................
Session 1: Technical Challenges with Carbon Fiber Manufacturing and Meeting End‐Product
Specifications for Vehicle Components from Bio‐Derived Materials ...................................................................................................
Session 2: Technical Challenges with Converting Biomass Resources to “Drop‐In” Carbon
Fiber Intermediates ...................................................................................................
Session 3: Unconventional Carbon Fiber—Resources, Challenges, and Opportunities ...................................................................................................
Concluding Remarks ...................................................................................................
Appendix A: Full Workshop Agenda ...................................................................................................
Appendix B: Workshop Questions ...................................................................................................
Appendix C: Abbreviations and Acronyms ...................................................................................................
Appendix D: Related Links ...................................................................................................
Appendix E: Workshop Attendees ...................................................................................................
Introduction
On June 4–5, 2013, the U.S. Department of Energy’s (DOE’s) Office of Energy Efficiency and Renewable Energy (EERE) hosted a workshop in Detroit, Michigan, to discuss research and development (R&D) related to the manufacturing of low‐cost carbon fiber from renewable resources that can be used for vehicle lightweighting.
Stakeholders from industry, national laboratories, and universities attended and provided valuable
information on topics ranging from the development of renewable feedstocks to the production of carbon fiber and its implementation in vehicle lightweighting.
Purpose, Need, and Process of the Workshop
The Clean Energy Manufacturing Initiative
DOE recently launched the Clean Energy Manufacturing Initiative1 to increase U.S. competitiveness in the production of clean‐energy products and enhance U.S. manufacturing across the board by increasing energy productivity. The Clean Energy Manufacturing Initiative focuses EERE's clean‐energy technology offices and Advanced Manufacturing Office around the competitive opportunity for the United States to be the leader in the clean‐energy manufacturing industries and jobs of both today and tomorrow. This initiative will bring together a wide array of relevant DOE and EERE offices, federal agencies, research institutions, and private-sector partners to map out and implement a strategy to ensure that U.S. manufacturers are competitive in the global marketplace.
DOE engages in multiple activities to enhance the Clean Energy Manufacturing Initiative, including dialogues and summits, requests for information, and investments in clean‐energy manufacturing. Through the Clean Energy Manufacturing Initiative, DOE’s Bioenergy Technologies Office, in coordination with the Advanced Manufacturing and Vehicle Technologies Offices, hosted the Renewable, Low‐Cost Carbon Fiber for Lightweight Vehicles Workshop. The purpose of the workshop was to leverage the complementary insight and expertise of EERE’s offices to support end‐to‐end technology development for an enhanced domestic supply chain for low‐cost carbon fiber that has the potential to increase energy productivity and the competitiveness of U.S. manufacturing.
Bioenergy Technologies Office
The availability of affordable feedstocks and robust supply chains are key to successful manufacturing industries. These characteristics are inherent to biomass; therefore, it has the potential to be a commercially viable and low‐cost renewable feedstock for the production of carbon fiber. Of all forms of renewable energy consumed in the United States, none rival the amount of energy produced from biomass.2 Indeed, the United States leads the world in the total size of cultivated land (approximately 1.7 million square kilometers)3 and the production of agricultural crops, such as soybean and maize. The United States also is the world‐leading producer of softwood and hardwood lumber. A recent report issued by DOE estimates a total renewable resource of 1 billion dry tons of agricultural residues, woody biomass, and new energy crops that can be
sustainably harvested every year.4 More than 130 million metric tons of crop residues alone exist today, which is more than sufficient to meet the current and anticipated growth in carbon fiber demand—even at imperfect conversion yields. Due to the relatively low energy density of unprocessed biomass, it is much more efficient to use the material near the source of production, which could advantage local production of carbon fiber or precursors compared to offshore production. It is the mission of EERE’s Bioenergy Technologies Office to transform the available domestic biomass resources into fuels, chemicals, and power.
Advanced Manufacturing Office
DOE’s Advanced Manufacturing Office supports R&D conducted at the Carbon Fiber Technology Facility
(CFTF)5 at Oak Ridge National Laboratory (ORNL). Through CFTF, ORNL is exploring the production of carbon fiber from different types of precursors, including lignin. ORNL has demonstrated significant progress toward the production of carbon fibers from lignin. Yet, there remain important manufacturing barriers to the deployment of lignin‐based carbon fibers in high-performance clean‐energy applications, such aslightweighting vehicles. Current barriers to the commercialization of lignin‐based carbon fiber include melt processability (especially for softwood lignins); long heat treatment time (especially for hardwood lignins); variability in the feedstock; need for purification and fractionation; inability to reach targets for strength and stiffness of completed fiber; and unproven scale of operation. Similar challenges are anticipated for other
precursor materials, such as textile‐based polyacrylonitrile (PAN) or polyolefins. A biobased process to make PAN precursor equivalent to the current commercially available material is likely to minimize some of these barriers (i.e., stabilization time), but will also present new processing challenges—such as the development of pure and high‐quality acrylonitrile chemicals. The Advanced Manufacturing Office remains interested in developing manufacturing processes for alternative precursor materials as a promising route for lowering the cost of carbon fiber and realizing the anticipated life‐cycle energy savings for manufactured products. AMO has also supported the development of conversion processes and manufacturing systems to produce carbon fiber‐reinforced composite products, in addition to performing extensive research, development, anddemonstration work in a wide range of manufacturing technologies.
Vehicle Technologies Office
Reducing the weight of vehicles is a strategic goal of EERE. Specifically, EERE’s Vehicle Technologies Office has been investigating material properties and manufacturing processes to bring about a new generation of lightweight materials, such as alternate carbon fiber precursors. The cost of incorporating carbon fiber composites in standard automobiles is a substantial issue. For widespread market adoption, it is estimated that the cost of PAN composites would need to reach $5 per pound or less.6 The impact of incorporating these lower‐cost composites would have significant positive effects. Lightweight materials could reduce passenger car weight by 50% and improve fuel efficiency by approximately 35% without compromising performance or safety.7 DOE estimates that through the strategic use of carbon fiber, automakers could cut the weight of cars by up to 70%.8 Even reducing vehicle weight by only 10% will result in a 4%–5% reduction in fuel use in passenger cars.9 Assuming that 8.8 million barrels of oil are consumed by passenger cars per day, a 10%
weight reduction in all passenger cars will result in an annual saving of more than 5 billion gallons of gasoline in the United States alone.
Current State of Technology
Current carbon fiber technology relies primarily on PAN chemical feedstock, which is derived from petroleum precursors. The cost of PAN can contribute up to 50% of the manufactured cost of carbon fiber.10 PAN is made by the free radical polymerization of acrylonitrile, typically produced by catalytic ammoxidation of propylene.
Propylene is often produced as a co‐product in petroleum refinery processes.11 As such, the precursor issubject to the price volatility of crude oil; as the cost of oil goes up, so does the cost of PAN. Industries that rely on or desire to use carbon fibers could experience a renaissance if a replacement for petroleum‐derived PAN could be found that offers competitive performance and is available at a lower cost. That resource can be biomass.
DOE is interested in enhanced collaboration between EERE offices to provide viable routes to manufacturing PAN from biomass‐derived chemical intermediates. Substitutes for PAN from renewable resources that are less expensive, less volatile in price, and have the same or better properties for the manufacture of carbon fiber are also under consideration for this collaboration.
Workshop Concept and Process
To attain the maximum benefit from the Renewable, Low‐Cost Carbon Fiber Workshop, DOE gathered knowledgeable subject matter experts with experience in carbon fiber manufacturing, biomass deconstruction and intermediate production, and industrial chemical production. These expert speakers provided information around the requirements of their respective industries and the areas where renewable carbon fiber would provide benefits. Issues discussed at the event included the technical challenges of carbon fiber manufacturing, including meeting end‐product specifications for vehicle structural components; the technical challenges of converting biomass resources to "drop‐in" carbon fiber intermediates; and the resources, challenges, and opportunities in unconventional carbon fiber.
Plenary talks given by DOE representatives highlighted the Department’s interest and activities in clean‐energy manufacturing and development of new technologies. These discussions featured the following speakers:
- Elizabeth (Libby) Wayman, DOE EERE Strategic Programs
- Neil Rossmeissl, DOE EERE Bioenergy Technologies Office
- Bhima Sastri, DOE EERE Advanced Manufacturing Office.
Subject matter experts with diverse experience in manufacturing, biomass, and vehicle components spoke about the requirements of their respective industries and areas where renewable carbon fiber could increase productivity and competitiveness. These discussions featured the following speakers:
- Gary Lownsdale, Plasan Carbon Composites
- Cliff Eberle and Mark Downing, Oak Ridge National Laboratory
- Darrell Waite, Old Town Fuel & Fiber
- Will Schroeder, ADM.
Participants were invited to answer a series of questions related to the development of renewable carbon fiber in breakout sessions (see Appendix B: Workshop Questions). Three breakouts were held, each addressing different questions and issues: Technical Challenges with Carbon Fiber Manufacturing and Meeting End‐Product Specifications for Vehicle Components from Bio‐Derived Materials; Technical Challenges with Converting Biomass Resources to “Drop‐In” Carbon Fiber Intermediates; and Unconventional Carbon Fiber: Resources, Challenges, and Opportunities. A full workshop agenda is provided in Appendix A. Breakout attendees were divided into three groups and asked specific questions related to the breakout discussion topic. The answers were recorded, and at the end of each breakout session, the comments weresummarized and reported to the group as a whole. The information gathered during those sessions is reported below.
Session 1: Technical Challenges with Carbon Fiber Manufacturing and Meeting End‐Product Specifications for Vehicle Components from Bio‐Derived Materials
A lignin pathway to carbon fibers has been researched by a number of different entities.12 Current
technologies do not yet yield a lignin‐derived carbon fiber with the tensile strength and modulus profiles required for the material to be incorporated into vehicle structural components. A route based on converting cellulosic sugars to PAN appears to offer a “drop‐in” route to functionally equivalent materials, which may be more readily commercialized in the near term. Cellulosic sugars can be converted by a combination of microbial and/or inorganic catalysts into a number of different monomers used for carbon fiber production today, including—but not limited to—acrylonitrile,13 methyl acrylate,14 and itaconic acid.15 The discussion for this report will focus largely on acrylonitrile, as it is the primary monomer for producing PAN‐based copolymers that are predominantly used as carbon fiber precursors.
A bio‐derived acrylonitrile would need to satisfy a number of different technical parameters to be adopted for use in existing manufacturing processes, namely polymerization and spinning, to generate PAN precursor fibers. Workshop participants identified three factors as critical to measure with respect to the acceptability of bio‐acrylonitrile—the yield from the raw feedstock (e.g., cellulosic sugars); the source, type, and quantity of chemical impurities; and the degree of pre‐polymerization.
Workshop participants expressed the view that if bio‐derived acrylonitrile can be made to be chemically and physically identical to petroleum‐derived acrylonitrile, then the existing downstream process should produce high‐quality PAN and carbon fiber. The functional equivalency of the bio‐PAN could be validated and established by measuring the bio‐acrylonitrile content, the appropriate reactivity with co‐monomers and polymerization catalysts, the resulting molecular weight distribution, the thermal oxidative stability, the uniformity and viscosity of the dope, and the ability to be spooled.
These critical technical performance factors can be evaluated by a number of different existing methods, including—but not limited to—analytical chemistry, thermogravimetric analysis, and other tests for material properties. The amount of material required for testing will vary depending on the step and stage of the overall manufacturing process and technology readiness, respectively. Early‐stage testing might require grams or kilograms of materials, and later manufacturing equivalency validation might require metric tons of materials. There is little, if any, tolerance for variability in these technical specifications if the intended end application is vehicle structural components. There may be higher tolerance for variability if the bio‐derived carbon fiber will be used for non‐structural components, so long as it is still produced cost-competitively to the alternatives.
Ultimately, bio‐acrylonitrile and bio‐PAN would need to meet existing American Society of Mechanical Engineers and American Society for Testing and Materials standards with manufacturing scaled‐up appropriately in order to gain market adoption. The production costs must be competitive with the existing process, especially if the bio‐PAN offers no other performance benefit. The bio‐derived PAN should seek to minimize the generation of hazardous or toxic byproducts and, overall, aim to have an improved environmental footprint in terms of energy use and material lifecycle (durability, recyclability). The future market volatility of fossil‐based PAN is an additional factor to consider in weighing the benefits and costs of a bio‐derived route.
Other raw materials used in the production of composites and other fiber precursors besides PAN can be bioderived. Some of the co‐monomers for this approach identified by participants include itaconic acid, methyl
acrylate, and vinyl acetate. Pitch, rayon, polyolefins, and bio‐oils were also identified as alternative precursors that can be sourced from biomass. Resin components, such as polyurethanes, for the carbon fiber composites offer an additional avenue for biobased chemicals. Functional equivalency or advantages will need to be established in each of these cases, but there are many more ways to incorporate biobased content for material applications.
Session 2: Technical Challenges with Converting Biomass Resources to “Drop‐In” Carbon Fiber Intermediates
Technical barriers of utilizing a biomass feedstock to produce drop‐in carbon fiber intermediates—such as acrylonitrile—include concerns about the supply chain, security and competitive biofuel fuel uses, regionalized supply, and overall availability. There is wide variability within biomass resources, and the barriers of measuring the purity of these feedstocks might not be well enough known for the applications that produce acrylonitrile and other bio‐derived intermediates for the carbon fiber industry. After a known feedstock can be reliably obtained and appropriately analyzed, there may be challenges within the biomass deconstruction processes for the production of carbon fiber intermediates. There will need to be strong communication between suppliers and customers on what specifications need to be met for the deconstructed biomass fractions, whether they are sugars or lignin. Workshop participants noted that it would be beneficial to have a biomass library that details the different sugar and lignin production from different types of raw biomass. Besides sugar and lignin, other intermediates for upgrading to bio‐derived acrylonitrile include carboxylic acids, sugar acids, amino acids, levulinic acid, glycerol, propylene, biocrude substitutes for refineries, and condensation products. Overall, there would need to be more crosscutting science between biomass feedstock scientists and polymer chemists/material engineers to better evaluate the potentials of biomass in the carbon fiber industry.
The upgrading of bio‐intermediates to acrylonitrile is not a straightforward process, and strategic R&D is needed to reach cost and yield parity with conventionally produced acrylonitrile. The supply and logistics of the biomass to the production facility, and the intermediates leaving the facility, would both require reliable supply and off‐take agreements. The CAPEX (capital expenditures) and OPEX (operating expense) of thescaling‐up of processes will require better catalyst efficiencies, among other improvements, to make renewable acrylonitrile cost‐competitive with current routes. Other than general economic concerns about the new conversion facility required for this process, the environmental issues that may arise from this are currently unfamiliar. Because toxicity of the waste streams is unknown, processing facilities for bio-acrylonitrile may require new environmental certifications, and the carbon efficiency and the release of carbon dioxide (CO2) and other potential greenhouse gases from these commercial‐scale processes have not yet been determined.
The supply chain barriers associated with bio‐derived acrylonitrile range from technical challenges to marketing hurdles. As mentioned previously, feedstock supply, logistics, storage, and stability will introduce a significant barrier in producing bio‐derived carbon fiber compared to conventionally derived carbon fiber. It may be beneficial if renewable carbon fiber facilities have the ability to utilize diversified biomass resources in order to help mitigate this risk. The commercial validation of these processes for fermenting complex sugars to potential acrylonitrile precursors—such as 3‐hydroxyproprionate, lactate, and amino acids—is still unproven, which heavily contributes to the challenges of bringing this technology to a commercial‐scale market.
Japan is currently the majority producer of carbon fiber worldwide, accounting for 37% of the supply.16 North America and Europe account for the majority of the demand for carbon fiber. The role of the United States in the competitive carbon fiber production market needs to be understood and established. There will need to be cost parity between international and national markets, and using biomass feedstocks appears to be a complex technical route. Additionally, when dealing with international imports/exports, export restrictions will need to be considered. In all of these main facets (technical, economic, political, and environmental), commercial production of bio‐derived carbon fiber will require collaborative efforts between diverse stakeholders. Many workshop participants stated that it may be beneficial to increase the knowledge base of bio‐derived carbon fiber by forming a consortium that will study and collaborate on the abovementioned wide range of barriers.
Session 3: Unconventional Carbon Fiber—Resources, Challenges, and Opportunities
Renewable carbon fiber derived from biomass may not need to be produced through a “drop‐in”
intermediate. Efforts to create unconventional carbon fiber would likely be longer term, as new processes and equipment would need to be developed. Previous work has sought to use lignin from pulp and paper mills as a feedstock for carbon fiber, with varying levels of success. Current cellulosic ethanol production requirements are leading to the generation of large amounts of lignin, which could be a potential carbon fiber feedstock. A more complete understanding of lignin, including knowledge of different lignin structures and their processability, would improve engineering efforts. Development of lignin‐derived carbon fiber would be improved with a catalogued library of lignin types, methods, and chemical and mechanical properties of the tested fibers. Work previously performed at ORNL could inform this library by summarizing what has been tried, what has succeeded, and what has failed, enabling advanced analysis of these results. A key understanding underpinning this information is the comparison of kraft lignin—produced from a chemical pulping process called kraft pulping—with lignin produced through other methods, such as those employed inthe existing and anticipated biofuels biorefineries. Technologies for biomass pretreatment for biofuels production may be able to tailor lignin structure or properties for carbon fiber production. DOE has funded work on lignin‐derived carbon fiber, and a review of those previously funded efforts would identify areas where enhanced or focused R&D could yield the greatest benefits.
In addition to lignin, cellulose from plants could be used to regenerate fibers in order to make carbon fiber—in a process similar to that of rayon. Other possible bio‐derived precursors that were mentioned during the workshop include fatty acids, olefins, pitch or rosin‐like substances, bio‐oils, and chitin.
Genetic modification of biomass may also enable new routes to carbon fiber, including engineering plants to synthesize lignin with properties more amenable to carbon fiber production, or other polymers that could be converted to PAN‐like materials. Participants suggested that carbon fibers could also be derived from CO2 or carbon nanotubes in yet‐to‐be‐developed methods.
Much of the previous work on bio‐derived carbon fiber has focused on lightweighting vehicles, particularly structural components. Some of the applications for vehicle lightweighting also include components like seat frames, door inner modules, mirror housings, air splitters, front wheel wells, roof inner panels, and bumper beams. It is possible that the properties of carbon fiber needed for other applications may not be as stringent; therefore, expanding the uses for bio‐derived carbon fiber. New carbon fiber types could be developed for coatings or in battery materials. Beyond these elements, insulation, flame‐retardant materials, dispersants for dyes, tires, interior appearance components, and audio systems could also be made from biomass‐derived carbon fiber. The long list of potential vehicle uses emphasizes a need for evaluating the specifications necessary for a variety of possible carbon fiber applications.
Carbon fiber use is not limited to vehicles—almost any industry can use a lower‐grade carbon fiber in place of existing materials. Many industries would benefit from lower‐cost carbon fiber that could be used as a substitute for existing materials; for example, marine vessels, road and bridge infrastructure, electrical transmission lines, flame proofing, medical applications, selective gas removal filters, fiberglass replacements, fabrics, sporting goods, and acoustics.
Workshop participants noted that the economic tradeoffs of new processes are important to understand. The development of bio‐derived carbon fiber will rely on understanding its economic value and tradeoffs relative to those of its petroleum‐derived counterparts. Lessons learned from petroleum‐derived carbon fiber could also be applied to a bio‐derived product to enhance and enable production. Finally, life‐cycle assessments will be crucial to understanding the benefits of renewable carbon fiber.
Concluding Remarks
DOE has a clear mandate to employ science and discovery that will not only reduce the United States’ dependence on foreign petroleum, but will also mitigate climate change associated with greenhouse gas emissions caused by the consumption of petroleum. Producing renewable carbon fiber from biomass can help fulfill this mandate by accomplishing the following:
- Developing the science and technology required to replace the entire barrel of oil, which includes the products made from petroleum.
- Achieving the higher margins associated with chemicals, which would enable the bioindustry.
- Lightweighting vehicles to increase fuel economy and efficiency while maintaining safety standards.
- Assisting U.S. industry by utilizing non‐fossil‐based feedstocks that are not subject to the price volatility associated with petroleum.
The focus of this workshop was to gather information from various stakeholders in an attempt to understand the R&D challenges that will need to be overcome in the production of a bio‐derived carbon fiber. DOE very much appreciates the input provided by industry, government, laboratory personnel, and academic researchers who made this report possible.
美国能源部:可再生低成本碳纤维制造研讨会总结报告

导语
2013年6月4-5日,美国能源部(DEO)下属的再生能源与能效办公室(EERE)在汽车城底特律举办了一场国际研讨会。会议以服务汽车轻量化为出发点,探讨了可再生、低成本碳纤维的研发和制造。参加会议的既有产业界的精英,也有学术界的权威专家。会议的主要议题包括如何寻找合适的可再生前驱体、如何将其应用于碳纤维生产并最终服务于汽车轻量化。
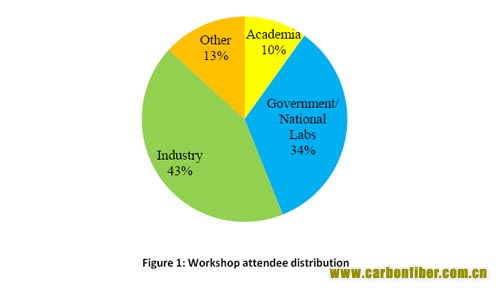
参会人员分类:产业界:43%;政府/国家实验室:34%;学校/研究院:10%;其他:13%
目的和议程
清洁能源生产动议书
美国能源部2013年7月间发布清洁能源生产动议书(Clean Energy Manufacturing Initiative),旨在提升美国在清洁能源生产领域的国际竞争力,进而借此提升美国制造产业的国际竞争力。该动议书由EERE下属的清洁能源技术办公室(Clean Energy Technology Office)和先进制造技术办公室(Advanced Manufacturing Office)负责具体操作,汇集了大批的产业界、学术界和政界的人员并机构,为美国制造业赢得未来市场的主动权谋划蓝图、制定战略。
为提升该动议书的影响力,美国能源部举办了一系列的座谈会、研讨会、咨询会,投资说明会等活动。作为系列会议之一,由能源部下属生物能源技术办公室(Bioenergy Technologies Office)与先进制造技术办公室(Advanced Manufacturing Office)、汽车技术办公室(Vehicle Technologies Office)合作举办了“汽车轻量化——可再生的低成本碳纤维制造”研讨会。研讨会的目的在于利用EERE及其下属各办公室的资源和专业优势,推动美国国内低成本碳纤维整个供应链条的技术进步,进而提升能源利用效率,提振美国制造业的国际竞争力。
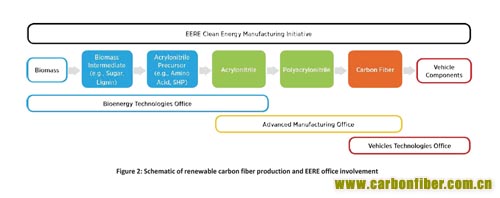
生物质资源——生物质中间体(糖类、木质素等)——丙烯腈前驱体(氨基酸、SHP等)——丙烯腈——聚丙烯腈——碳纤维——碳纤维汽车零部件
生物能源技术办公室(Bioenergy Technologies Office)
找到成本适宜、加工技术成熟的原材料(feedstock),并建立灵活稳定的供应链条是制造业成功的关键。对于碳纤维制造业来说,生物质资源(Biomass)充分满足上述条件,是一种可用以商业化运作的低成本原材料。在美国使用的所有可再生能源中,生物质能源毫无疑问是产量最大的一个。美国拥有世界上最大的耕地面积(约170万平方公里),其大豆、玉米灯农作物产量高居世界首位。美国同时是世界上最大的软木和硬木生产商。美国能源部最新发布的报告显示,全美农、林业生产剩余物(residue)等可再生生物资源多达10亿吨(干重),其中仅农作物生产剩余物一项(超过1.3亿吨),即便资源得不到充分转化,也远远超过碳纤维生产当今以及短期未来的需要。由于未经加工的生物质资源能量密度(Energy Density)较低,比较经济的方法是就近利用,实现碳纤维或其原丝的本地化生产。生物能源技术办公室的职责就在于想办法将国内可利用的生物质资源有效地转化成燃料、化学产品和电力能源。
先进制造技术办公室(Advanced Manufacturing Office)
该办公室的职能在于为橡树岭国家实验室(Oak Ridge National Laboratory, ORNL)的碳纤维技术试验生产线(Carbon Fiber Technology Facility, CFTF)提供研发支持。在CFTF中,研究人员主要从事包括木质素在内的多途径碳纤维前驱体制造技术的开发研究。单就木质素这一途径而言,ORNL已经取得了不小的进展。然而,在诸如汽车轻量化等具体应用中,使用木质素基碳纤维还存在一定的生产障碍。现如今想要实现木质素基碳纤维的商业化生产之前必须先解决以下几点困难:1. 熔融加工性能(Melt-Processability,尤其是软木木质素);2. 过长的热处理时间(尤其是硬木木质素);3. 原料(feedstock)庞杂,需要提纯(Purification)和分馏(Fractionation);4. 成丝后强度和硬度达不到预期指标;5. 规模量产可到什么程度未经验证。而其他前驱体的制备也存在类似的问题,比如说聚烯烃(Polyofelins)。以生物质资源为途径生产当今商业使用的PAN原丝的同等品,能使得上述障碍中的部分最小化,但是又会带了新的问题,好比说如何保证丙烯腈(Acrylonitrile)的纯度和质量。先进制造技术办公室的职能就是推动研发不同种前驱体材料的制备技术、降低产品生命周期内的能源消耗。该办公室同样推动研发能源转化技术和碳纤维制品的制造技术。
汽车技术办公室(Vehicle Technologies Office)
汽车轻量化是EERE的重要战略目标。汽车技术办公室的职能在于研究分析材料性能和制备工艺、推动研发新一代轻质材料。将碳纤维推广到量产车生产是一个耗资巨大的工程。据推算,只有当其使用成本降低到5美元/磅或以下的时候才可能实现。有鉴于此,研发出低成本的碳纤维制备方法将大大加快这一进程。轻质材料将使车重减轻50%,能耗降低35%左右,而其包括安全在内的各项性能并不受重量减轻的影响。美国能源部预测,使用碳纤维最多可减轻车重70%。即便车重仅仅降低10%,都会带来能耗4%-5%降低的红利。如今,小汽车每天要消耗880万桶石油。所有车重减轻10%,仅在美国每天就可以节约超过50亿加仑的汽油。
当下的技术状况
当下的碳纤维制备主要依赖PAN原丝,而PAN原丝炼自石油。PAN原丝的制备成本占到碳纤维整个制备成本的50%。石油精炼后的副产品丙烯(Propylene)经过催化氨氧化反应(Catalytic Ammoxidation)生成丙烯腈,再经过自由基聚合(Free Radical Polymerization)之后生成PAN原丝。因此,PAN原丝的价格受制于原油价格的波动,彼涨此涨。如若能找到PAN原丝的替代产品,碳纤维的使用成本必回大大下降。这种替代产品就是生物质资源。而美国能源部正在极力促成下属各办公室之间的合作来寻找这种合适的PAN原丝替代品。
研讨会的进程
为了让与会者有尽可能大的收获,美国能源部邀请了在碳纤维制造、生物质资源处理、中间体制备以及工业化学品生产领域经验丰富的专家学者发表演讲。这些专家学者将从各自专业领域的角度分析使用可再生碳纤维所需的满足的条件以及可能带来的好处。讲演的主题涵盖了碳纤维制备的技术挑战、应用于汽车零部件生产需要满足的条件、将生物质资源转化成碳纤维制备中间体材料的技术挑战以及非PAN基碳纤维带来的种种机遇等等。
在全体人员会议上,美国能源部将着重强调其对清洁能源制造技术和其他新技术的兴趣,介绍人包括:
·战略规划项目组 Elizabeth(Libby)Wayman
·生物能源技术办公室 Neil Rossmeissl
·先进制造技术办公室 Bhima Sastri
发表主题演讲的各领域专家学者包括:
·Plasan Carbon Composites公司 Gary Lownsdale
·橡树岭国家实验室 Cliff Eberle 和 Mark Downing
·Old Town Fuel & Fiber公司 Darrell Waite
·ADM公司 Will Schroeder
此外,研讨会还就三个议题分别设立了子会议。这是议题分别是:1. 碳纤维制备的技术挑战以及生物源材料制备汽车零部件需要满足的条件;2. 利用生物质资源生产低成本碳纤维中间体的技术挑战;3. 突破传统的碳纤维:资源、挑战和机遇。整个研讨会的时程安排详见原文。在子会议中,与会者将被分为三个小组,就一系列问题展开讨论(问题列表详见原文)。讨论内容将被记录、汇总并形成书面报告。
与会名单

注释
Plasan Carbon Composites公司:美国碳纤维汽车零部件生产企业
Old Town Fuel & Fiber公司:美国纸浆生产企业
ADM公司:世界最大的小麦、玉米及油籽加工企业之一
赞助单位:美国能源部 再生能源与能效办公室
生物能源技术办公室
报告整理:Joyce Yang Katy Christiansen Sarah Luchner
编译:中国复材展组委会
Renewable, Low‐Cost Carbon Fiber for Lightweight Vehicles
Summary Report from the June 4–5, 2013, Workshop
Detroit, MI
Workshop and Summary Report sponsored by the
U.S. Department of Energy
Office of Energy Efficiency and Renewable Energy
Bioenergy Technologies Office
Summary report edited by Joyce Yang, Katy Christiansen, and Sarah Luchner
Preface
The U.S. Department of Energy’s (DOE’s) Office of Energy Efficiency and Renewable Energy (EERE) invests in a diverse portfolio of energy technologies to achieve a stronger economy, a cleaner environment, and a more secure energy future for America.
This report summarizes the results of a public workshop sponsored by DOE/EERE in Detroit, Michigan, on June 4–5, 2013. The views and opinions of the workshop attendees, as summarized in this document, do not necessarily reflect those of the United States government or any agency thereof, or do their employees make any warranty, expressed or implied, or assume any liability or responsibility for the accuracy, completeness, or usefulness of any information, apparatus, product, or process disclosed, or represent that its use would not infringe privately owned rights.
Table of Contents
Introduction ...................................................................................................
Purpose, Need, and Process of the Workshop ...................................................................................................
Session 1: Technical Challenges with Carbon Fiber Manufacturing and Meeting End‐Product
Specifications for Vehicle Components from Bio‐Derived Materials ...................................................................................................
Session 2: Technical Challenges with Converting Biomass Resources to “Drop‐In” Carbon
Fiber Intermediates ...................................................................................................
Session 3: Unconventional Carbon Fiber—Resources, Challenges, and Opportunities ...................................................................................................
Concluding Remarks ...................................................................................................
Appendix A: Full Workshop Agenda ...................................................................................................
Appendix B: Workshop Questions ...................................................................................................
Appendix C: Abbreviations and Acronyms ...................................................................................................
Appendix D: Related Links ...................................................................................................
Appendix E: Workshop Attendees ...................................................................................................
Introduction
On June 4–5, 2013, the U.S. Department of Energy’s (DOE’s) Office of Energy Efficiency and Renewable Energy (EERE) hosted a workshop in Detroit, Michigan, to discuss research and development (R&D) related to the manufacturing of low‐cost carbon fiber from renewable resources that can be used for vehicle lightweighting.
Stakeholders from industry, national laboratories, and universities attended and provided valuable
information on topics ranging from the development of renewable feedstocks to the production of carbon fiber and its implementation in vehicle lightweighting.
Purpose, Need, and Process of the Workshop
The Clean Energy Manufacturing Initiative
DOE recently launched the Clean Energy Manufacturing Initiative1 to increase U.S. competitiveness in the production of clean‐energy products and enhance U.S. manufacturing across the board by increasing energy productivity. The Clean Energy Manufacturing Initiative focuses EERE's clean‐energy technology offices and Advanced Manufacturing Office around the competitive opportunity for the United States to be the leader in the clean‐energy manufacturing industries and jobs of both today and tomorrow. This initiative will bring together a wide array of relevant DOE and EERE offices, federal agencies, research institutions, and private-sector partners to map out and implement a strategy to ensure that U.S. manufacturers are competitive in the global marketplace.
DOE engages in multiple activities to enhance the Clean Energy Manufacturing Initiative, including dialogues and summits, requests for information, and investments in clean‐energy manufacturing. Through the Clean Energy Manufacturing Initiative, DOE’s Bioenergy Technologies Office, in coordination with the Advanced Manufacturing and Vehicle Technologies Offices, hosted the Renewable, Low‐Cost Carbon Fiber for Lightweight Vehicles Workshop. The purpose of the workshop was to leverage the complementary insight and expertise of EERE’s offices to support end‐to‐end technology development for an enhanced domestic supply chain for low‐cost carbon fiber that has the potential to increase energy productivity and the competitiveness of U.S. manufacturing.
Bioenergy Technologies Office
The availability of affordable feedstocks and robust supply chains are key to successful manufacturing industries. These characteristics are inherent to biomass; therefore, it has the potential to be a commercially viable and low‐cost renewable feedstock for the production of carbon fiber. Of all forms of renewable energy consumed in the United States, none rival the amount of energy produced from biomass.2 Indeed, the United States leads the world in the total size of cultivated land (approximately 1.7 million square kilometers)3 and the production of agricultural crops, such as soybean and maize. The United States also is the world‐leading producer of softwood and hardwood lumber. A recent report issued by DOE estimates a total renewable resource of 1 billion dry tons of agricultural residues, woody biomass, and new energy crops that can be
sustainably harvested every year.4 More than 130 million metric tons of crop residues alone exist today, which is more than sufficient to meet the current and anticipated growth in carbon fiber demand—even at imperfect conversion yields. Due to the relatively low energy density of unprocessed biomass, it is much more efficient to use the material near the source of production, which could advantage local production of carbon fiber or precursors compared to offshore production. It is the mission of EERE’s Bioenergy Technologies Office to transform the available domestic biomass resources into fuels, chemicals, and power.
Advanced Manufacturing Office
DOE’s Advanced Manufacturing Office supports R&D conducted at the Carbon Fiber Technology Facility
(CFTF)5 at Oak Ridge National Laboratory (ORNL). Through CFTF, ORNL is exploring the production of carbon fiber from different types of precursors, including lignin. ORNL has demonstrated significant progress toward the production of carbon fibers from lignin. Yet, there remain important manufacturing barriers to the deployment of lignin‐based carbon fibers in high-performance clean‐energy applications, such aslightweighting vehicles. Current barriers to the commercialization of lignin‐based carbon fiber include melt processability (especially for softwood lignins); long heat treatment time (especially for hardwood lignins); variability in the feedstock; need for purification and fractionation; inability to reach targets for strength and stiffness of completed fiber; and unproven scale of operation. Similar challenges are anticipated for other
precursor materials, such as textile‐based polyacrylonitrile (PAN) or polyolefins. A biobased process to make PAN precursor equivalent to the current commercially available material is likely to minimize some of these barriers (i.e., stabilization time), but will also present new processing challenges—such as the development of pure and high‐quality acrylonitrile chemicals. The Advanced Manufacturing Office remains interested in developing manufacturing processes for alternative precursor materials as a promising route for lowering the cost of carbon fiber and realizing the anticipated life‐cycle energy savings for manufactured products. AMO has also supported the development of conversion processes and manufacturing systems to produce carbon fiber‐reinforced composite products, in addition to performing extensive research, development, anddemonstration work in a wide range of manufacturing technologies.
Vehicle Technologies Office
Reducing the weight of vehicles is a strategic goal of EERE. Specifically, EERE’s Vehicle Technologies Office has been investigating material properties and manufacturing processes to bring about a new generation of lightweight materials, such as alternate carbon fiber precursors. The cost of incorporating carbon fiber composites in standard automobiles is a substantial issue. For widespread market adoption, it is estimated that the cost of PAN composites would need to reach $5 per pound or less.6 The impact of incorporating these lower‐cost composites would have significant positive effects. Lightweight materials could reduce passenger car weight by 50% and improve fuel efficiency by approximately 35% without compromising performance or safety.7 DOE estimates that through the strategic use of carbon fiber, automakers could cut the weight of cars by up to 70%.8 Even reducing vehicle weight by only 10% will result in a 4%–5% reduction in fuel use in passenger cars.9 Assuming that 8.8 million barrels of oil are consumed by passenger cars per day, a 10%
weight reduction in all passenger cars will result in an annual saving of more than 5 billion gallons of gasoline in the United States alone.
Current State of Technology
Current carbon fiber technology relies primarily on PAN chemical feedstock, which is derived from petroleum precursors. The cost of PAN can contribute up to 50% of the manufactured cost of carbon fiber.10 PAN is made by the free radical polymerization of acrylonitrile, typically produced by catalytic ammoxidation of propylene.
Propylene is often produced as a co‐product in petroleum refinery processes.11 As such, the precursor issubject to the price volatility of crude oil; as the cost of oil goes up, so does the cost of PAN. Industries that rely on or desire to use carbon fibers could experience a renaissance if a replacement for petroleum‐derived PAN could be found that offers competitive performance and is available at a lower cost. That resource can be biomass.
DOE is interested in enhanced collaboration between EERE offices to provide viable routes to manufacturing PAN from biomass‐derived chemical intermediates. Substitutes for PAN from renewable resources that are less expensive, less volatile in price, and have the same or better properties for the manufacture of carbon fiber are also under consideration for this collaboration.
Workshop Concept and Process
To attain the maximum benefit from the Renewable, Low‐Cost Carbon Fiber Workshop, DOE gathered knowledgeable subject matter experts with experience in carbon fiber manufacturing, biomass deconstruction and intermediate production, and industrial chemical production. These expert speakers provided information around the requirements of their respective industries and the areas where renewable carbon fiber would provide benefits. Issues discussed at the event included the technical challenges of carbon fiber manufacturing, including meeting end‐product specifications for vehicle structural components; the technical challenges of converting biomass resources to "drop‐in" carbon fiber intermediates; and the resources, challenges, and opportunities in unconventional carbon fiber.
Plenary talks given by DOE representatives highlighted the Department’s interest and activities in clean‐energy manufacturing and development of new technologies. These discussions featured the following speakers:
- Elizabeth (Libby) Wayman, DOE EERE Strategic Programs
- Neil Rossmeissl, DOE EERE Bioenergy Technologies Office
- Bhima Sastri, DOE EERE Advanced Manufacturing Office.
Subject matter experts with diverse experience in manufacturing, biomass, and vehicle components spoke about the requirements of their respective industries and areas where renewable carbon fiber could increase productivity and competitiveness. These discussions featured the following speakers:
- Gary Lownsdale, Plasan Carbon Composites
- Cliff Eberle and Mark Downing, Oak Ridge National Laboratory
- Darrell Waite, Old Town Fuel & Fiber
- Will Schroeder, ADM.
Participants were invited to answer a series of questions related to the development of renewable carbon fiber in breakout sessions (see Appendix B: Workshop Questions). Three breakouts were held, each addressing different questions and issues: Technical Challenges with Carbon Fiber Manufacturing and Meeting End‐Product Specifications for Vehicle Components from Bio‐Derived Materials; Technical Challenges with Converting Biomass Resources to “Drop‐In” Carbon Fiber Intermediates; and Unconventional Carbon Fiber: Resources, Challenges, and Opportunities. A full workshop agenda is provided in Appendix A. Breakout attendees were divided into three groups and asked specific questions related to the breakout discussion topic. The answers were recorded, and at the end of each breakout session, the comments weresummarized and reported to the group as a whole. The information gathered during those sessions is reported below.
Session 1: Technical Challenges with Carbon Fiber Manufacturing and Meeting End‐Product Specifications for Vehicle Components from Bio‐Derived Materials
A lignin pathway to carbon fibers has been researched by a number of different entities.12 Current
technologies do not yet yield a lignin‐derived carbon fiber with the tensile strength and modulus profiles required for the material to be incorporated into vehicle structural components. A route based on converting cellulosic sugars to PAN appears to offer a “drop‐in” route to functionally equivalent materials, which may be more readily commercialized in the near term. Cellulosic sugars can be converted by a combination of microbial and/or inorganic catalysts into a number of different monomers used for carbon fiber production today, including—but not limited to—acrylonitrile,13 methyl acrylate,14 and itaconic acid.15 The discussion for this report will focus largely on acrylonitrile, as it is the primary monomer for producing PAN‐based copolymers that are predominantly used as carbon fiber precursors.
A bio‐derived acrylonitrile would need to satisfy a number of different technical parameters to be adopted for use in existing manufacturing processes, namely polymerization and spinning, to generate PAN precursor fibers. Workshop participants identified three factors as critical to measure with respect to the acceptability of bio‐acrylonitrile—the yield from the raw feedstock (e.g., cellulosic sugars); the source, type, and quantity of chemical impurities; and the degree of pre‐polymerization.
Workshop participants expressed the view that if bio‐derived acrylonitrile can be made to be chemically and physically identical to petroleum‐derived acrylonitrile, then the existing downstream process should produce high‐quality PAN and carbon fiber. The functional equivalency of the bio‐PAN could be validated and established by measuring the bio‐acrylonitrile content, the appropriate reactivity with co‐monomers and polymerization catalysts, the resulting molecular weight distribution, the thermal oxidative stability, the uniformity and viscosity of the dope, and the ability to be spooled.
These critical technical performance factors can be evaluated by a number of different existing methods, including—but not limited to—analytical chemistry, thermogravimetric analysis, and other tests for material properties. The amount of material required for testing will vary depending on the step and stage of the overall manufacturing process and technology readiness, respectively. Early‐stage testing might require grams or kilograms of materials, and later manufacturing equivalency validation might require metric tons of materials. There is little, if any, tolerance for variability in these technical specifications if the intended end application is vehicle structural components. There may be higher tolerance for variability if the bio‐derived carbon fiber will be used for non‐structural components, so long as it is still produced cost-competitively to the alternatives.
Ultimately, bio‐acrylonitrile and bio‐PAN would need to meet existing American Society of Mechanical Engineers and American Society for Testing and Materials standards with manufacturing scaled‐up appropriately in order to gain market adoption. The production costs must be competitive with the existing process, especially if the bio‐PAN offers no other performance benefit. The bio‐derived PAN should seek to minimize the generation of hazardous or toxic byproducts and, overall, aim to have an improved environmental footprint in terms of energy use and material lifecycle (durability, recyclability). The future market volatility of fossil‐based PAN is an additional factor to consider in weighing the benefits and costs of a bio‐derived route.
Other raw materials used in the production of composites and other fiber precursors besides PAN can be bioderived. Some of the co‐monomers for this approach identified by participants include itaconic acid, methyl
acrylate, and vinyl acetate. Pitch, rayon, polyolefins, and bio‐oils were also identified as alternative precursors that can be sourced from biomass. Resin components, such as polyurethanes, for the carbon fiber composites offer an additional avenue for biobased chemicals. Functional equivalency or advantages will need to be established in each of these cases, but there are many more ways to incorporate biobased content for material applications.
Session 2: Technical Challenges with Converting Biomass Resources to “Drop‐In” Carbon Fiber Intermediates
Technical barriers of utilizing a biomass feedstock to produce drop‐in carbon fiber intermediates—such as acrylonitrile—include concerns about the supply chain, security and competitive biofuel fuel uses, regionalized supply, and overall availability. There is wide variability within biomass resources, and the barriers of measuring the purity of these feedstocks might not be well enough known for the applications that produce acrylonitrile and other bio‐derived intermediates for the carbon fiber industry. After a known feedstock can be reliably obtained and appropriately analyzed, there may be challenges within the biomass deconstruction processes for the production of carbon fiber intermediates. There will need to be strong communication between suppliers and customers on what specifications need to be met for the deconstructed biomass fractions, whether they are sugars or lignin. Workshop participants noted that it would be beneficial to have a biomass library that details the different sugar and lignin production from different types of raw biomass. Besides sugar and lignin, other intermediates for upgrading to bio‐derived acrylonitrile include carboxylic acids, sugar acids, amino acids, levulinic acid, glycerol, propylene, biocrude substitutes for refineries, and condensation products. Overall, there would need to be more crosscutting science between biomass feedstock scientists and polymer chemists/material engineers to better evaluate the potentials of biomass in the carbon fiber industry.
The upgrading of bio‐intermediates to acrylonitrile is not a straightforward process, and strategic R&D is needed to reach cost and yield parity with conventionally produced acrylonitrile. The supply and logistics of the biomass to the production facility, and the intermediates leaving the facility, would both require reliable supply and off‐take agreements. The CAPEX (capital expenditures) and OPEX (operating expense) of thescaling‐up of processes will require better catalyst efficiencies, among other improvements, to make renewable acrylonitrile cost‐competitive with current routes. Other than general economic concerns about the new conversion facility required for this process, the environmental issues that may arise from this are currently unfamiliar. Because toxicity of the waste streams is unknown, processing facilities for bio-acrylonitrile may require new environmental certifications, and the carbon efficiency and the release of carbon dioxide (CO2) and other potential greenhouse gases from these commercial‐scale processes have not yet been determined.
The supply chain barriers associated with bio‐derived acrylonitrile range from technical challenges to marketing hurdles. As mentioned previously, feedstock supply, logistics, storage, and stability will introduce a significant barrier in producing bio‐derived carbon fiber compared to conventionally derived carbon fiber. It may be beneficial if renewable carbon fiber facilities have the ability to utilize diversified biomass resources in order to help mitigate this risk. The commercial validation of these processes for fermenting complex sugars to potential acrylonitrile precursors—such as 3‐hydroxyproprionate, lactate, and amino acids—is still unproven, which heavily contributes to the challenges of bringing this technology to a commercial‐scale market.
Japan is currently the majority producer of carbon fiber worldwide, accounting for 37% of the supply.16 North America and Europe account for the majority of the demand for carbon fiber. The role of the United States in the competitive carbon fiber production market needs to be understood and established. There will need to be cost parity between international and national markets, and using biomass feedstocks appears to be a complex technical route. Additionally, when dealing with international imports/exports, export restrictions will need to be considered. In all of these main facets (technical, economic, political, and environmental), commercial production of bio‐derived carbon fiber will require collaborative efforts between diverse stakeholders. Many workshop participants stated that it may be beneficial to increase the knowledge base of bio‐derived carbon fiber by forming a consortium that will study and collaborate on the abovementioned wide range of barriers.
Session 3: Unconventional Carbon Fiber—Resources, Challenges, and Opportunities
Renewable carbon fiber derived from biomass may not need to be produced through a “drop‐in”
intermediate. Efforts to create unconventional carbon fiber would likely be longer term, as new processes and equipment would need to be developed. Previous work has sought to use lignin from pulp and paper mills as a feedstock for carbon fiber, with varying levels of success. Current cellulosic ethanol production requirements are leading to the generation of large amounts of lignin, which could be a potential carbon fiber feedstock. A more complete understanding of lignin, including knowledge of different lignin structures and their processability, would improve engineering efforts. Development of lignin‐derived carbon fiber would be improved with a catalogued library of lignin types, methods, and chemical and mechanical properties of the tested fibers. Work previously performed at ORNL could inform this library by summarizing what has been tried, what has succeeded, and what has failed, enabling advanced analysis of these results. A key understanding underpinning this information is the comparison of kraft lignin—produced from a chemical pulping process called kraft pulping—with lignin produced through other methods, such as those employed inthe existing and anticipated biofuels biorefineries. Technologies for biomass pretreatment for biofuels production may be able to tailor lignin structure or properties for carbon fiber production. DOE has funded work on lignin‐derived carbon fiber, and a review of those previously funded efforts would identify areas where enhanced or focused R&D could yield the greatest benefits.
In addition to lignin, cellulose from plants could be used to regenerate fibers in order to make carbon fiber—in a process similar to that of rayon. Other possible bio‐derived precursors that were mentioned during the workshop include fatty acids, olefins, pitch or rosin‐like substances, bio‐oils, and chitin.
Genetic modification of biomass may also enable new routes to carbon fiber, including engineering plants to synthesize lignin with properties more amenable to carbon fiber production, or other polymers that could be converted to PAN‐like materials. Participants suggested that carbon fibers could also be derived from CO2 or carbon nanotubes in yet‐to‐be‐developed methods.
Much of the previous work on bio‐derived carbon fiber has focused on lightweighting vehicles, particularly structural components. Some of the applications for vehicle lightweighting also include components like seat frames, door inner modules, mirror housings, air splitters, front wheel wells, roof inner panels, and bumper beams. It is possible that the properties of carbon fiber needed for other applications may not be as stringent; therefore, expanding the uses for bio‐derived carbon fiber. New carbon fiber types could be developed for coatings or in battery materials. Beyond these elements, insulation, flame‐retardant materials, dispersants for dyes, tires, interior appearance components, and audio systems could also be made from biomass‐derived carbon fiber. The long list of potential vehicle uses emphasizes a need for evaluating the specifications necessary for a variety of possible carbon fiber applications.
Carbon fiber use is not limited to vehicles—almost any industry can use a lower‐grade carbon fiber in place of existing materials. Many industries would benefit from lower‐cost carbon fiber that could be used as a substitute for existing materials; for example, marine vessels, road and bridge infrastructure, electrical transmission lines, flame proofing, medical applications, selective gas removal filters, fiberglass replacements, fabrics, sporting goods, and acoustics.
Workshop participants noted that the economic tradeoffs of new processes are important to understand. The development of bio‐derived carbon fiber will rely on understanding its economic value and tradeoffs relative to those of its petroleum‐derived counterparts. Lessons learned from petroleum‐derived carbon fiber could also be applied to a bio‐derived product to enhance and enable production. Finally, life‐cycle assessments will be crucial to understanding the benefits of renewable carbon fiber.
Concluding Remarks
DOE has a clear mandate to employ science and discovery that will not only reduce the United States’ dependence on foreign petroleum, but will also mitigate climate change associated with greenhouse gas emissions caused by the consumption of petroleum. Producing renewable carbon fiber from biomass can help fulfill this mandate by accomplishing the following:
- Developing the science and technology required to replace the entire barrel of oil, which includes the products made from petroleum.
- Achieving the higher margins associated with chemicals, which would enable the bioindustry.
- Lightweighting vehicles to increase fuel economy and efficiency while maintaining safety standards.
- Assisting U.S. industry by utilizing non‐fossil‐based feedstocks that are not subject to the price volatility associated with petroleum.
The focus of this workshop was to gather information from various stakeholders in an attempt to understand the R&D challenges that will need to be overcome in the production of a bio‐derived carbon fiber. DOE very much appreciates the input provided by industry, government, laboratory personnel, and academic researchers who made this report possible.